Click on image for the answer
Saturday, 5 November 2011
Monday, 4 July 2011
Wednesday, 29 June 2011
Monday, 20 June 2011
Wednesday, 1 June 2011
Kyoto Protocol participation map
Labels:
Kyoto
Tuesday, 31 May 2011
Friday, 27 May 2011
Saturday, 21 May 2011
Sea ice volume since 1979
![]() |
Arctic sea ice volume by month in cubic kilometers (with simple quadratic trend lines projecting to zero volume, details here). The bottom (red) line is September volume. |
Thursday, 19 May 2011
Tuesday, 10 May 2011
Tuesday, 3 May 2011
Monday, 28 March 2011
Sunday, 27 March 2011
Monday, 21 March 2011
Thursday, 17 March 2011
Wednesday, 16 March 2011
Tuesday, 1 March 2011
A Journey through Climate History
A fantastic timeline showing key events in the climatic history of the planet, alongside events in human history.
The Epochs are Deep Time - 4.5 Billion Years Ago, Holocene - 1750 to Last 10,000 Years, Industrial - 1900 to 1750 and Modern - 2009 to 1900.
The four scenarios for the future are possible ways in which societies could evolve as we move through the century.
Saturday, 26 February 2011
Distributions and ranges for climate sensitivity from different lines of evidence
![]() |
Distributions and ranges for climate sensitivity from different lines of evidence. The circle indicates the most likely value. The thin colored bars indicate very likely value (more than 90% probability). The thicker colored bars indicate likely values (more than 66% probability). Dashed lines indicate no robust constraint on an upper bound. The IPCC likely range (2 to 4.5°C) and most likely value (3°C) are indicated by the vertical grey bar and black line, respectively (Knutti and Hegerl 2008) |
Melting Snow and Ice Warm Northern Hemisphere
The left image shows how much energy the NorthernHemisphere’s snow and ice—called the cryosphere—reflected on average between 1979 and 2008. Dark blue indicates more reflected energy, in Watts per square meter, and thus more cooling. The Greenland ice sheet reflects more energy than any other single location in the Northern Hemisphere. The second-largest contributor to cooling is the cap of sea ice over the Arctic Ocean.
The right image shows how the energy being reflected from the cryosphere has changed between 1979 and 2008. When snow and ice disappear, they are replaced by dark land or ocean, both of which absorb energy. The image shows that the Northern Hemisphere is absorbing more energy, particularly along the outer edges of the Arctic Ocean, where sea ice has disappeared, and in the mountains of Central Asia.
Climate Tipping Points
![]() |
Probability distribution for the committed warming by GHGs between 1750 and 2005 from Ramanathan and Feng (2008). The normalized distribution is calculated from the probability density function given by Roe and Baker (2007), and the mean and standard deviation of the uncertainties associated with feedback processes are fitted for Sanderson et al. (2007). Shown are the climate-tipping elements and the temperature threshold range that initiates the tipping. |
Friday, 25 February 2011
Monday, 21 February 2011
Global Temperature Relative to 1800-1900
![]() |
Northern Hemisphere proxy temperature reconstruction (purple - Mann et al. 2008) vs. the instrumental temperature record (black) and projected 21st Century surface temperature changes in various IPCC emissions scenarios (red, yellow, green). Source: Copenhagen Diagnosis. |
Global Temperature Model
![]() |
Global surface temperature record (black - NASA GISS) with one-sigma error bars (blue) and model runs for the IPCC A1B emissions scenario (red, yellow). |
Friday, 18 February 2011
GHCN Monthly / Australia's high quality climate change dataset.
A great deal of effort went into the homogeneity adjustments. Yet the effects of the homogeneity adjustments on global average temperature trends are minor (Easterling and Peterson 1995b). However, on scales of half a continent or smaller, the homogeneity adjustments can have an impact. On an individual time series, the effects of the adjustments can be enormous. These adjustments are the best we could do given the paucity of historical station history metadata on a global scale. But using an approach based on a reference series created from surrounding stations means that the adjusted station's data is more indicative of regional climate change and less representative of local microclimatic change than an individual station not needing adjustments.
A change in the type of thermometer shelter used at many Australian observation sites in the early 20th century resulted in a sudden drop in recorded temperatures which is entirely spurious. It is for this reason that these early data are currently not used for monitoring climate change. Other common changes at Australian sites over time include location moves, construction of buildings or growth of vegetation around the observation site and, more recently, the introduction of Automatic Weather Stations.
The impacts of these changes on the data are often comparable in size to real climate variations, so they need to be removed before long-term trends are investigated. Procedures to identify and adjust for non-climatic changes in historical climate data generally involve a combination of:
Ø investigating historical information (metadata) about the observation site,
Ø using statistical tests to compare records from nearby locations, and
Ø using comparison data recorded simultaneously at old and new locations, or with old and new instrument types.
Full details of the procedure are described here
Thursday, 10 February 2011
Hydrological Changes from IPCC Fourth Assessment Report: Climate Change 2007: Working Group I: The Physical Science Basis
Tuesday, 8 February 2011
NOAA State of the Climate 2010
- For 2010, the combined global land and ocean surface temperature tied with 2005 as the warmest such period on record, at 0.62°C (1.12°F) above the 20th century average of 13.9°C (57.0°F). 1998 is the third warmest year-to-date on record, at 0.60°C (1.08°F) above the 20th century average.
- The 2010 Northern Hemisphere combined global land and ocean surface temperature was the warmest year on record, at 0.73°C (1.31°F) above the 20th century average. The 2010 Southern Hemisphere combined global land and ocean surface temperature was the sixth warmest year on record, at 0.51°C (0.92°F) above the 20th century average.
- The global land surface temperature for 2010 tied with 2005 as the second warmest on record, at 0.96°C (1.73°F) above the 20th century average. The warmest such period on record occurred in 2007, at 0.99°C (1.78°F) above the 20th century average.
- The global ocean surface temperature for 2010 tied with 2005 as the third warmest on record, at 0.49°C (0.88°F) above the 20th century average.
- In 2010 there was a dramatic shift in the El Niño–Southern Oscillation, which influences temperature and precipitation patterns around the world. A moderate-to-strong El Niño at the beginning of the year transitioned to La Niña conditions by July. At the end of November, La Niña was moderate-to-strong.
Friday, 4 February 2011
Friday, 28 January 2011
How La Nina triggered floods in Australia
Thursday, 27 January 2011
Monday, 24 January 2011
Thursday, 20 January 2011
Wednesday, 19 January 2011
Thursday, 13 January 2011
Wednesday, 12 January 2011
How do we know more CO2 is causing warming?

Figure 1: Carbon dioxide concentrations in the atmosphere over both the last 1000 years and the preceding 400,000 years as measured in ice cores
As a greenhouse gas, this increase in atmospheric CO2 increases the amount of downward longwave radiation from the atmosphere, including towards the Earth's surface.
Surface measurements of downward longwave radiation
The increase in atmospheric CO2 and other greenhouse gases has increased the amount of infrared radiation absorbed and re-emitted by these molecules in the atmosphere. The Earth receives energy from the Sun in the form of visible light and ultraviolet radiation, which is then re-radiated away from the surface as thermal radiation in infrared wavelengths. Some of this thermal radiation is then absorbed by greenhouse gases in the atmosphere and re-emitted in all directions, some back downwards, increasing the amount of energy bombarding the Earth's surface. This increase in downward infrared radiation has been observed through spectroscopy, which measures changes in the electromagnetic spectrum.
Figure 2: Spectrum of the greenhouse radiation measured at the surface. Greenhouse effect from water vapor is filtered out, showing the contributions of other greenhouse gases (Evans 2006).
Satellite measurements of outgoing longwave radiation
The increased greenhouse effect is also confirmed by NASA's IRIS satellite and the Japanese Space Agency's IMG satellite observing less longwave leaving the Earth's atmosphere.
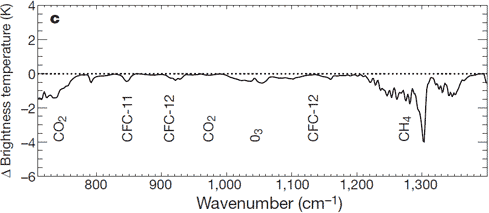
Figure 3: Change in spectrum from 1970 to 1996 due to trace gases. 'Brightness temperature' indicates equivalent blackbody temperature (Harries 2001).
The increased energy reaching the Earth's surface from the increased greenhouse effect causes it to warm. So how do we quantify the amount of warming that it causes?
Radiative Transfer Models
Radiative transfer models use fundamental physical equations and observations to translate this increased downward radiation into a radiative forcing, which effectively tells us how much increased energy is reaching the Earth's surface. Studies have shown that these radiative transfer models match up with the observed increase in energy reaching the Earth's surface with very good accuracy (Puckrin 2004). Scientists can then derive a formula for calculating the radiative forcing based on the change in the amount of each greenhouse gas in the atmosphere (Myhre 1998). Each greenhouse gas has a different radiative forcing formula, but the most important is that of CO2:
dF = 5.35 ln(C/Co)
Where 'dF' is the radiative forcing in Watts per square meter, 'C' is the concentration of atmospheric CO2, and 'Co' is the reference CO2 concentration. Normally the value of Co is chosen at the pre-industrial concentration of 280 ppmv.
Now that we know how to calculate the radiative forcing associated with an increase in CO2, how do we determine the associated temperature change?
Climate sensitivity
As the name suggests, climate sensitivity is an estimate of how sensitive the climate is to an increase in a radiative forcing. The climate sensitivity value tells us how much the planet will warm or cool in response to a given radiative forcing change. As you might guess, the temperature change is proportional to the change in the amount of energy reaching the Earth's surface (the radiative forcing), and the climate sensitivity is the coefficient of proportionality:
dT = λ*dF
Where 'dT' is the change in the Earth's average surface temperature, 'λ' is the climate sensitivity, usually with units in Kelvin or degrees Celsius per Watts per square meter (°C/[W/m2]), and 'dF' is the radiative forcing.
So now to calculate the change in temperature, we just need to know the climate sensitivity. Studies have given a possible range of values of 2-4.5°C warming for a doubling of CO2 (IPCC 2007). Using these values it's a simple task to put the climate sensitivity into the units we need, using the formulas above:
λ = dT/dF = dT/(5.35 * ln[2])= [2 to 4.5°C]/3.7 = 0.54 to 1.2°C/(W/m2)
Using this range of possible climate sensitivity values, we can plug λ into the formulas above and calculate the expected temperature change. The atmospheric CO2concentration as of 2010 is about 390 ppmv. This gives us the value for 'C', and for 'Co' we'll use the pre-industrial value of 280 ppmv.
dT = λ*dF = λ * 5.35 * ln(390/280) = 1.8 * λ
Plugging in our possible climate sensitivity values, this gives us an expected surface temperature change of about 1–2.2°C of global warming, with a most likely value of 1.4°C. However, this tells us the equilibrium temperature. In reality it takes a long time to heat up the oceans due to their thermal inertia. For this reason there is currently a planetary energy imbalance, and the surface has only warmed about 0.8°C. In other words, even if we were to immediately stop adding CO2 to the atmosphere, the planet would warm another ~0.6°C until it reached this new equilibrium state (confirmed by Hansen 2005). This is referred to as the 'warming in the pipeline'.
Of course this is just the temperature change we expect to observe from the CO2 radiative forcing. Humans cause numerous other radiative forcings, both positive (e.g. other greenhouse gases) and negative (e.g. sulfate aerosols which block sunlight). Fortunately, the negative and positive forcings are roughly equal and cancel each other out, and the natural forcings over the past half century have also been approximately zero (Meehl 2004), so the radiative forcing from CO2 alone gives us a good estimate as to how much we expect to see the Earth's surface temperature change.
Figure 4: Radiative forcing estimates from the IPCC report
We can also calculate the most conservative possible temperature change in response to the CO2 increase. Some climate scientists who are touted as 'skeptics' have suggested the actual climate sensitivity could be closer to 1°C for a doubling of CO2, or 0.27°C/(W/m2). Although numerous studies have ruled out climate sensitivity values this low, it's worth calculating how much of a temperature change this unrealistically low value would generate. Using the same formulas as above,
dT = 1.8 * λ = 1.8 * 0.27 = 0.5°C.
Therefore, even under this ultra-conservative unrealistic low climate sensitivity scenario, the increase in atmospheric CO2 over the past 150 years would account for over half of the observed 0.8°C increase in surface temperature.
From skepticalscience.com
Thursday, 6 January 2011
Wednesday, 5 January 2011
Monday, 3 January 2011
Subscribe to:
Posts (Atom)